Why is Threose Nucleic Acid (TNA) modification being used?
The use of oligonucleotides in therapeutics and diagnostics is rapidly expanding, particularly in the realm of antisense therapy. In previous discussions, we explored how modifying the backbone of antisense oligonucleotides (ASOs) with Mesyl can enhance stability and therapeutic efficacy. In this blog, we shift our focus to a promising class of artificial genetic polymers known as Xeno-nucleic acids (XNAs), with specific emphasis on α-L-Threose nucleic acid (TNA). TNA has attracted significant interest due to its structural simplicity and unique ability to form stable duplex structures with complementary DNA and RNA strands.
Various studies have investigated the biological properties and potential of TNA as antisense agents, including inhibition of GFP gene expression, detection of target miRNAs, and real-time monitoring of miRNA expression levels in cell lines [3,4,5]. Results indicate that TNA exhibits excellent resistance to acid degradation, high stability at room temperature storage, low cytotoxicity, and significant uptake in various cell lines without carriers. While further studies are necessary to fully validate the benefits of TNA, it remains an attractive candidate for biomedical and therapeutic applications.
What is Threose Nucleic Acid (TNA) modification and how is it synthesized?
TNA, or threose nucleic acid, represents a synthetic analog of DNA and RNA, featuring threofuranosyl nucleotides and a backbone comprised of threose sugar units, threofuranose, instead of the ribose or deoxyribose typically found in DNA and RNA. Unlike the conventional 3′- to 5′-linkage in DNA and RNA, the phosphate group in TNA connects at the 2′ to 3′-carbon. To synthesize TNA oligonucleotides, TNA phosphoramidite monomers of adenine (A), guanine (G), cytosine (C), and thymine (T)/uracil (U)) are utilized. The synthesis process follows Eschenmoser’s procedure, involving glycosylation, debenzoylation, tritylation, and phosphitylation steps. After stable pre-drying, the monomers are dissolved in acetonitrile and utilized in the solid-phase synthesis method using controlled pore glass. To ensure high purity and yield, the frequency of de-blocking and coupling reactions is increased, along with the reaction time in each synthesis cycle. Post-synthesis purification is conducted using C18 reverse-phase HPLC or conventional polyacrylamide gel electrophoresis (PAGE), with purity and molecular weight analysis confirmed by MALDI-TOF MS.
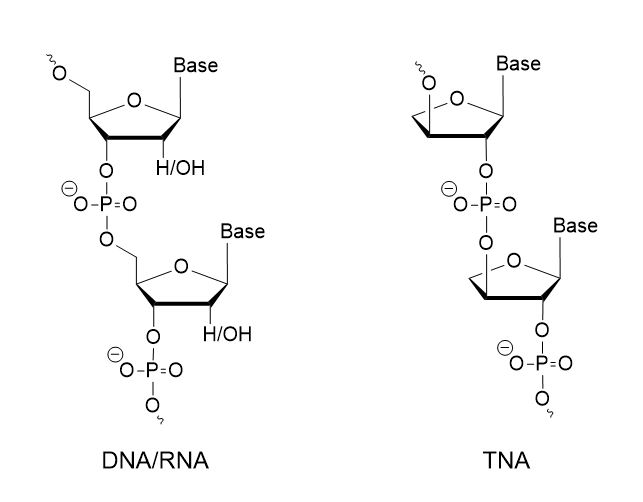
Does Synoligo synthesize TNA?
Yes, we have over 500+ modifications you can choose from for your project including TNA and TNA-U (Bz).
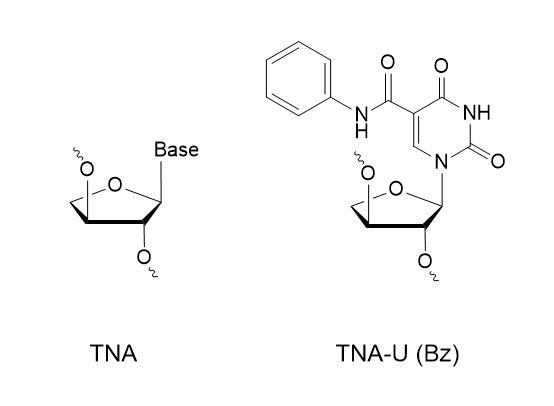
Feel free to contact us if you have any questions about starting a project with us.
References:
- Zhang, S., & Chaput, J. C. (2012). Synthesis of Threose Nucleic Acid (TNA) Phosphoramidite Monomers and Oligonucleotide Polymers. Current Protocols in Nucleic Acid Chemistry, 4.51.1–4.51.26. doi:10.1002/0471142700.nc0451s50
- Bege, M.; Borbás, A. The Medicinal Chemistry of Artificial Nucleic Acids and Therapeutic Oligonucleotides. Pharmaceuticals 2022, 15, 909. https://doi.org/10.3390/ph15080909
- Liu, L. S., Leung, H. M., Tam, D. Y., Lo, T. W., Wong, S. W., & Lo, P. K. (2018). α-l-Threose Nucleic Acids as Biocompatible Antisense Oligonucleotides for Suppressing Gene Expression in Living Cells. ACS Applied Materials & Interfaces, 10(11), 9736–9743. doi:10.1021/acsami.8b01180
- Wang, F., Liu, L. S., Li, P., Lau, C. H., Leung, H. M., Chin, Y. R., Tin, C., & Lo, P. K. (2022). Cellular uptake, tissue penetration, biodistribution, and biosafety of threose nucleic acids: Assessing in vitro and in vivo delivery. Materials today. Bio, 15, 100299. https://doi.org/10.1016/j.mtbio.2022.100299
- Wang, F., Liu, L. S., Li, P., Leung, H. M., Tam, D. Y., & Lo, P. K. (2022). Biologically stable threose nucleic acid-based probes for real-time microrna detection and imaging in living cells. Molecular Therapy – Nucleic Acids, 27, 787–796. https://doi.org/10.1016/j.omtn.2021.12.040